Understanding the Absence of Dark Matter Detection
Written on
The detection of dark matter remains elusive, but this absence of evidence doesn't diminish the possibility of its existence. One might liken the search for dark matter to looking for the number 3 between 1 and 2 — it simply isn't there, yet that doesn't imply it doesn't exist elsewhere.
Imagine you propose a theory that suggests additional particles or interactions are at play in our universe, potentially solving some of the fundamental enigmas in natural sciences. You would begin by crafting a hypothesis, outlining its predictions, and identifying observable outcomes.
Some outcomes are model-independent, meaning they would manifest regardless of the correctness of a particular theory, while others are model-dependent, appearing only under specific theoretical frameworks. When dark matter experiments yield no results, they primarily assess these model-dependent theories, leaving the model-independent implications unchallenged. Thus, their emptiness does not provide definitive proof against dark matter's existence.
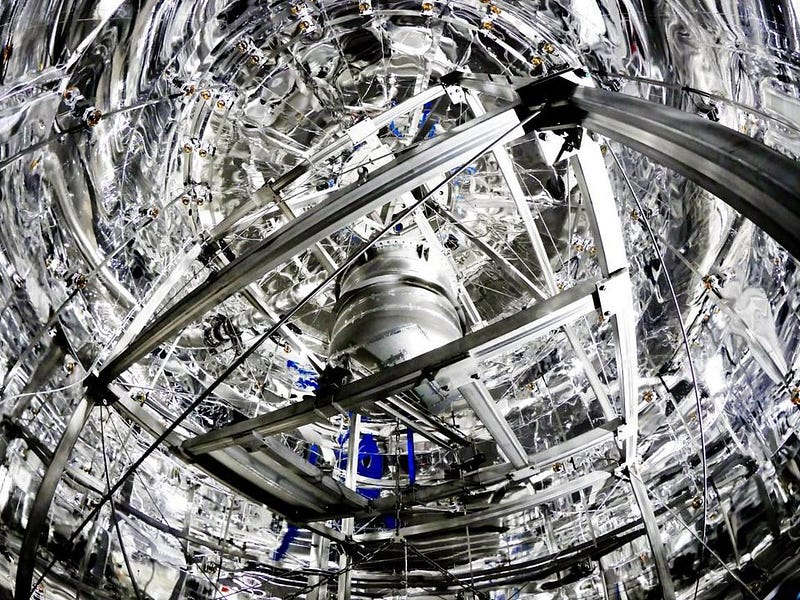
The quest for dark matter is driven by the hope that nature might yield fruitful results from improbable endeavors. Many groundbreaking discoveries have arisen from unexpected circumstances, prompting scientists to pursue low-cost yet high-reward experiments. This is the essence behind direct dark matter searches.
To explore the potential for dark matter detection, one must first consider the extensive knowledge already available. This foundational evidence guides us in assessing the likelihood of direct detection. While we haven't yet observed dark matter interacting with another particle directly, the indirect evidence strongly suggests its existence.
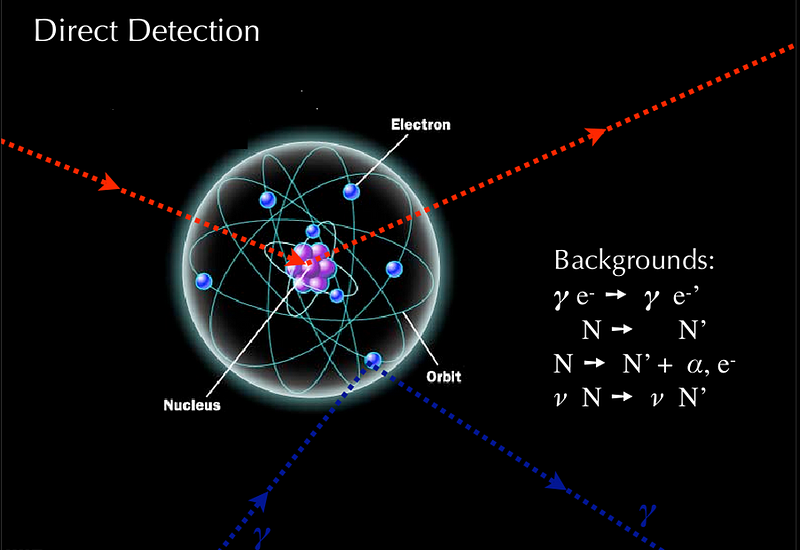
All known particles of the Standard Model have been directly observed, including the Higgs Boson. These particles, which include protons, neutrons, and electrons, constitute the matter we recognize in the universe, while other particles, such as quarks and gluons, form even more fundamental components. The concept of dark matter posits that additional, unknown particles significantly contribute to the universe's total matter content, a revolutionary idea that may seem far-fetched.
The rationale for considering dark matter arises from an examination of the universe itself. Scientific inquiry has revealed a wealth of information about distant celestial bodies, leading to well-established understandings of stellar functions and gravitational laws. When we analyze galaxies and their clusters, two essential conclusions emerge:
- Mass estimation of structures: By studying the motions and gravitational interactions of celestial bodies, we can estimate the total mass within these systems.
- Mass in stars: Our knowledge of stellar physics allows us to quantify the mass contained within stars based on their emitted light.
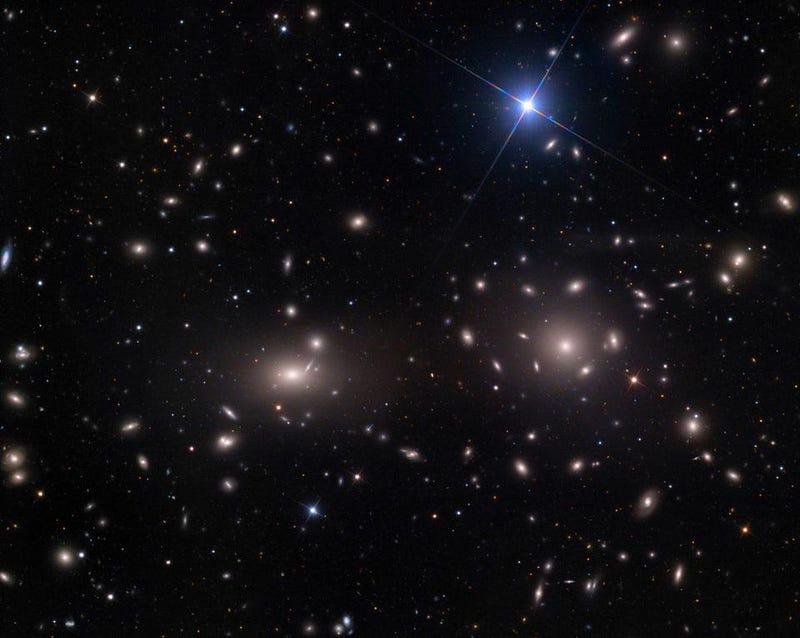
These two mass estimates yield a staggering discrepancy, differing by a factor of around 50. Clearly, something beyond visible stars must account for the majority of matter in the universe, a concept that compels further investigation into dark matter, supported by a wealth of observational evidence.
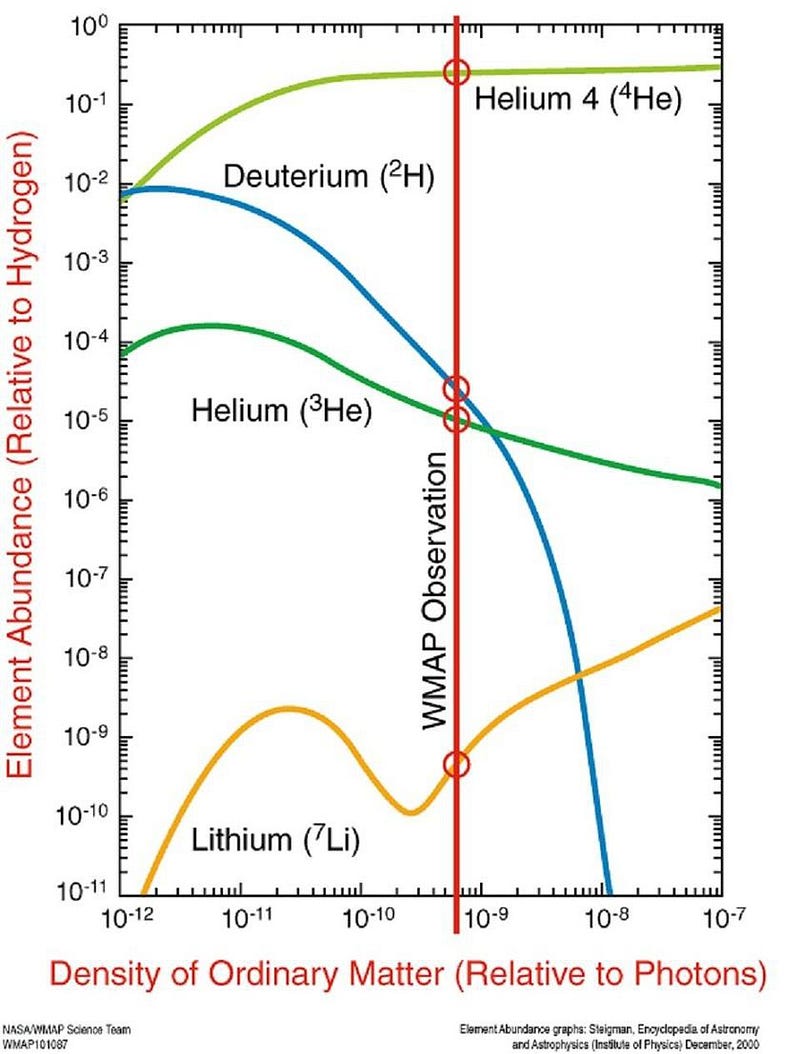
When we trace the physics laws back to the early universe, we find a time when conditions were too extreme for even nuclei to form. However, as the universe cooled, light nuclei, including isotopes of hydrogen and helium, emerged. The Big Bang Nucleosynthesis accurately estimates the total quantity of “normal matter” present, revealing it to be about one-sixth of the mass inferred from gravitational interactions, indicating that normal matter alone cannot explain the total mass observed.
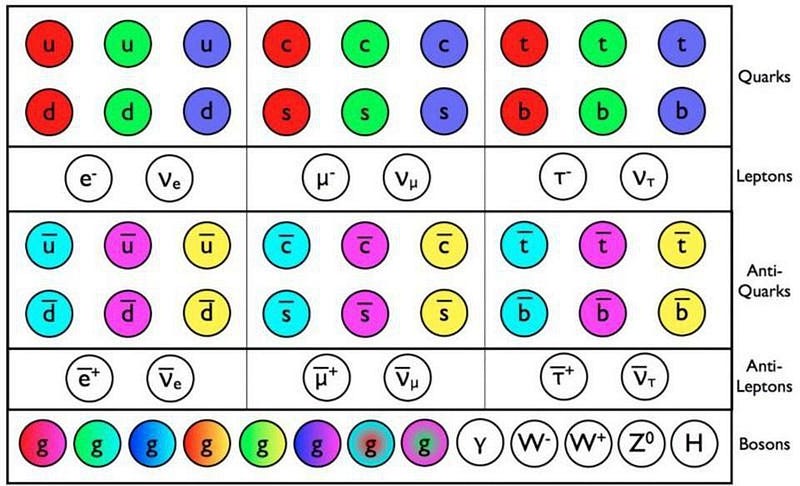
Further evidence arises from the Cosmic Microwave Background (CMB), which provides insights into the universe's early conditions. The CMB fluctuations indicate the proportions of normal and dark matter, consistently suggesting that dark matter constitutes approximately five-sixths of all matter.
Observations of baryon acoustic oscillations at large scales also suggest that the universe is dominated by dark matter, with only a small fraction attributed to normal matter. This conclusion is reinforced by the visible cosmic web, where gravitational forces shape matter into clusters and structures based on initial density fluctuations.
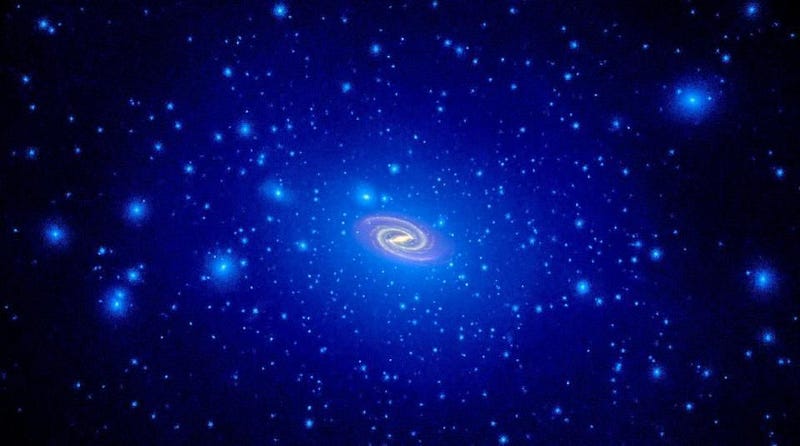
In summary, every galaxy and cluster is expected to be surrounded by a vast, diffuse halo of dark matter, exhibiting minimal interactions with normal matter. Despite the countless dark matter particles passing through our bodies and the Earth undetected, their elusive nature complicates direct detection.
Fortunately, indirect detection methods, such as gravitational lensing, offer promising avenues. By observing the distortion of light from distant galaxies caused by intervening mass, we can infer the presence and quantity of dark matter. Collisions between galaxy clusters further illuminate this phenomenon, showcasing the separation of normal matter and dark matter post-collision.
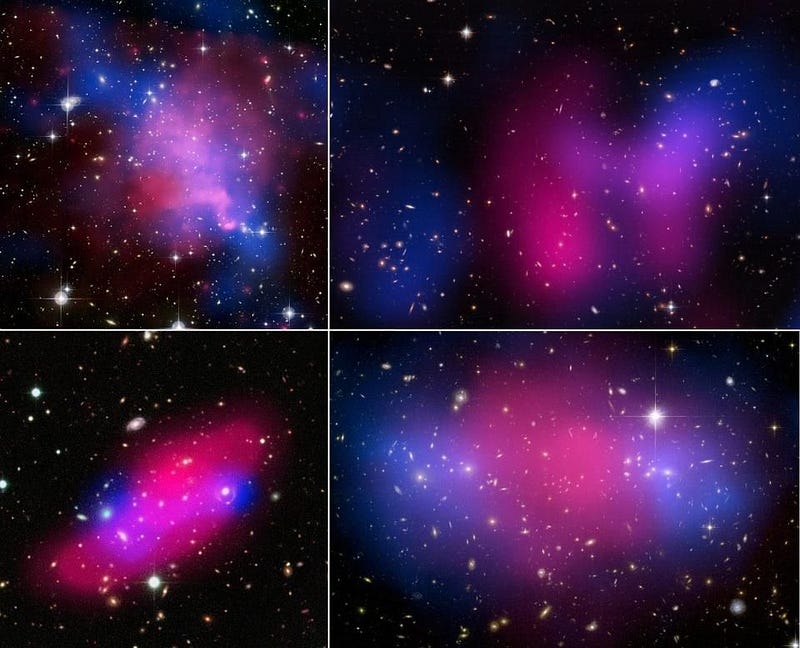
While we have yet to achieve direct detection of dark matter, the existing model-independent evidence presents a compelling case for its existence. Despite numerous efforts yielding no conclusive results, the quest for dark matter continues, driven by the recognition that absence of evidence does not equate to evidence of absence. In this pursuit of cosmic knowledge, we must remain vigilant and open-minded, as the mysteries of the universe await discovery.
Starts With A Bang is now featured on Forbes and republished on Medium thanks to our Patreon supporters. Ethan has authored two books, Beyond The Galaxy and Treknology: The Science of Star Trek from Tricorders to Warp Drive.